Ever heard of the idea that when you lose a sense, your other senses become markedly better to make up for the loss? Individuals with sensory impairment are often able to make remarkable adjustments to everyday tasks in order to carry on living as usual. In some cases, however, people develop what seem to be superpowers.
One example is the case of human echolocation expert, Daniel Kish. Kish, who lost his eyesight as an infant, is able to use a series of high-pitched clicks and echoes to navigate the world [1]. By listening for the differences in the acoustic signature of the clicks’ echoes as they rebound off objects and surfaces, he is able navigate and even distinguish between objects around him [2]. This technique, which he calls “Flashsonar”, is essentially how animals such as bats are able to navigate in the dark [3].
But Kish himself says that he doesn’t have “superhuman” hearing – in fact, Kish actively teaches Flashsonar to help other blind and vision-impaired individuals [2].
Like many other interesting phenomena in neuroscience, these “superhuman” senses might be explained, in part, by neuroplasticity.
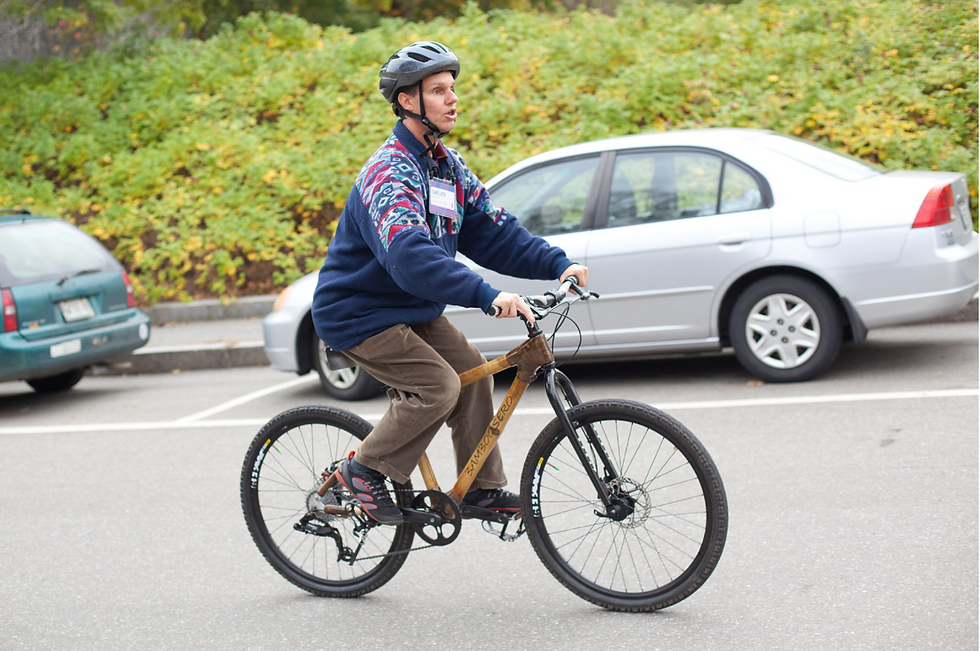
Figure 1. Human echolocation allows vision-impaired individuals to live independently. Kish (above) is able to navigate and even ride bikes with the help of echolocation [4].
The Power of Neuroplasticity
Neuroplasticity is the nervous system's ability to adapt in response to either internal or external stimuli by reorganising its neural structure with new neurons or modifying transmission at the synapses [5].
In the case of sensory loss, neuroplasticity can help the nervous system adapt by allowing the unused sensory cortex of the lost sense to process other stimuli through cross-modal reorganisation. For example, researchers working with C. elgans, a species of roundworm instrumental as a model for understanding biology, found that turning off the “touch neurons” of these worms creates “super-smellers” - individuals capable of detecting much fainter odours of food than control worms with an intact sense of touch [6].
In humans, researchers have found that the posterior superior temporal gyrus (STG), a region processing auditory stimuli, was activated by visual motion in deaf individuals, as seen in Figure 2, with increased activity in this region associated with decreased hearing aid use [7]. Similarly, one study of Braille readers using fMRI found that the visual cortex in the occipital and occipital-temporal regions was recruited during this task, even in participants who lost vision later on in life [8]. Whilst researchers weren’t exactly sure why these regions are activated, the results suggest they are either involved in processing both visual and tactile language, or that these Braille readers are using the visual cortex in a novel way [8].
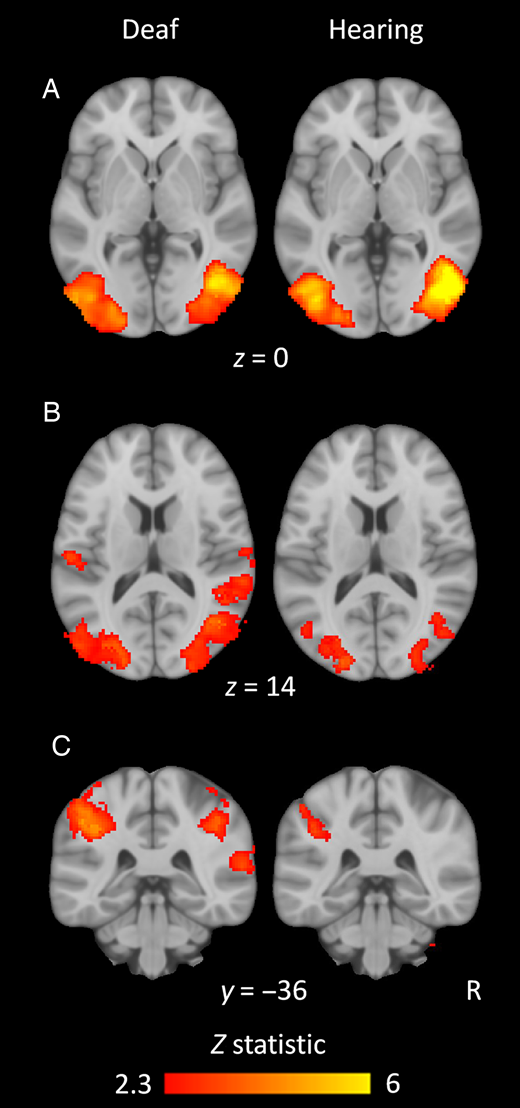
Figure 2. Both deaf and hearing groups showed similar activity in regions typically associated with processing visual motion when presented with moving visual stimuli (A). However, the visual stimuli also activated brain regions typically associated with auditory stimuli, with more significant activation in the posterior STG and intraparietal sulcus deaf group compared to the hearing group in (B) and (C). In this figure, y and z values refer to the coordinates the image was taken at, with the Z statistic used to compare activation across the MRIs of different subjects [7].
This type of cross-modal reorganisation of the visual cortex has actually been demonstrated in fMRI scans of Kish, although interestingly, this reorganisation was not seen in other echolocators in the same study who learned the skill at a much later age [9]. This is potentially related to Kish having taught himself echolocation during his “critical” or “sensitive” period of development, when his occipital cortex was potentially more “neuroplastic” and more sensitive to integration with other sensory modalities [9].
But it isn’t necessarily that these expert echolocators like Kish can hear more – instead, studies show that blind participants have better “spectral” and “temporal resolution” when it comes to sound, meaning that they are better than sighted participants at detecting differences in pitch, source and changes in sound [10].
Other researchers have also found that subjects with sensory impairment perform similarly to control subjects when assessing the acuity of an individual sense [11]. However, potentially due to increased selective attention and practise, they often perform better than controls in specific skills - for example, vision-impaired individuals demonstrate better auditory memory than sighted counterparts [11].
So rather than saying that they’ve developed actual “super-senses”, perhaps we should consider individuals with sensory impairment as being more skilled. And perhaps in ordinary individuals, our senses are just not working to their maximum all the time [12].
“Super-skill” vs “Super-sense”
But if “super-senses” are a skill, could we all improve our senses?
The idea of harnessing selective attention has actually been trialled in the restaurant world through a trend called “dining in the dark”, first offered by Blindekuh in Zurich [13]. Aside from fostering dialogue between sighted patrons and vision-impaired staff members, one line of thought behind this is that if you can’t see the food, you focus more on other senses like smell, texture (touch) and of course, taste [14].
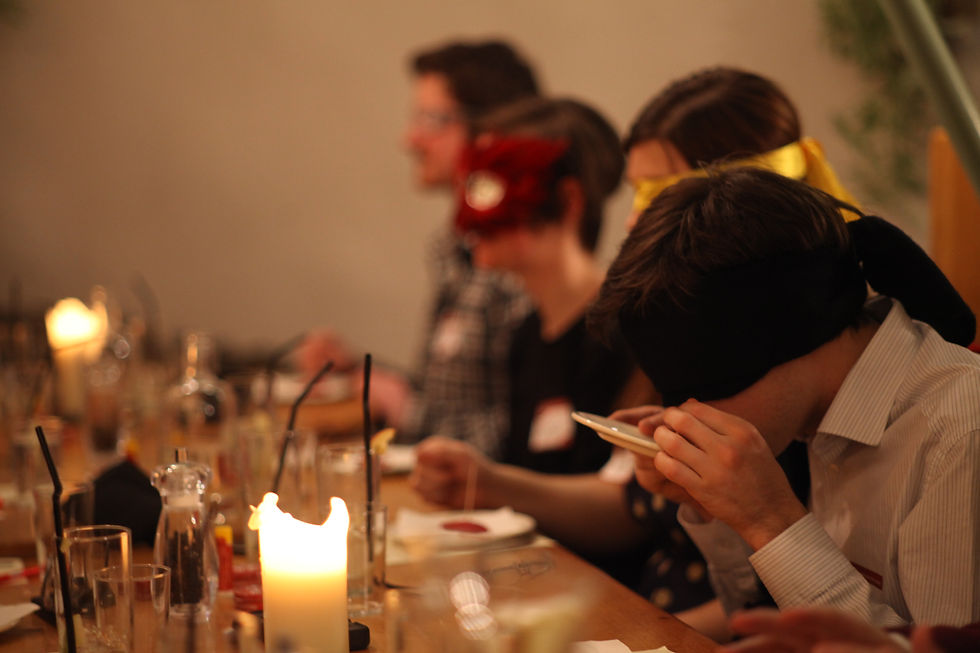
Figure 3. Aside from helping to spark empathetic conversations about how the blind community navigates the world, it is thought that “dining in the dark” (above) might help diners gain a greater appreciation for food by forcing them to focus on other senses such as smell and taste [15].
Although scientists are not actually sure if this really works [14], it goes to show that there might be other ways to boost your senses without the need for a plastic brain.
Neuroplasticity – Where to Next?
Although cross-modal neuroplasticity sounds like a great adaptation, neuroplasticity isn’t always helpful for individuals struggling with sensory impairment. For senses like vision, the brain has a critical (or sensitive) period during early development where it is the most “plastic” [16]. Reorganisation of the visual cortex during this critical period has meant that untreated vision problems in early life can lead to permanent impairment, even if the condition is treated later on [16].
But there are solutions available on the horizon. Recent research suggests that the brain still retains a bit of neuroplastic potential even after the critical period has passed, and that this potential can be reactivated or enhanced through pharmacological means or by manipulating sensory and motor experiences [14]. In the case of human echolocation, some studies have actually found both sighted and blind participants were able to learn the skill and their degree of improvement wasn’t limited by either their age or degree of blindness [17]. This means that this skill has the potential to help improve independence, even if they are at an earlier stage of progressive vision loss.
Whether neuroplasticity is a help or a hindrance, there’s still so much that we don’t know about how our brains function and about all the amazing ways that individuals can adapt to sensory impairment. In the meantime, regardless of its accuracy, these incredible skills truly deserve to be called a “super-sense”.
References:
[1] Chinnery H. Sensory Compensation in Children Following Vision Loss after Trauma and Disease. Journal of Clinical Research and Ophthalmology. 2015;2:049-53.
[2] Kish D. Experience: I taught myself to see. The Guardian. 2013.
[3] Thaler L, Norman L. Learning How to Be a Human Bat. Scientific American. 2017.
[4] Cook, T. “Daniel Kish - PopTech 2011 - Camden Maine USA” .Poptech. 2011. Available from: https://www.flickr.com/photos/40287103@N07/6270648236
[5] Mateos-Aparicio P, Rodríguez-Moreno A. The Impact of Studying Brain Plasticity. Frontiers in Cellular Neuroscience. 2019;13(66).
[6] Rabinowitch I, Laurent P, Zhao B, Walker D, Beets I, Schoofs L, et al. Neuropeptide-Driven Cross-Modal Plasticity following Sensory Loss in Caenorhabditis elegans. PLOS Biology. 2016;14(1):e1002348.
[7] Shiell MM, Champoux F, Zatorre RJ. Reorganization of Auditory Cortex in Early-deaf People: Functional Connectivity and Relationship to Hearing Aid Use. Journal of Cognitive Neuroscience. 2015;27(1):150-63.
[8] Burton H, Snyder AZ, Conturo TE, Akbudak E, Ollinger JM, Raichle ME. Adaptive Changes in Early and Late Blind: A fMRI Study of Braille Reading. Journal of Neurophysiology. 2002;87(1):589-607.
[9] Arnott SR, Thaler L, Milne JL, Kish D, Goodale MA. Shape-specific activation of occipital cortex in an early blind echolocation expert. Neuropsychologia. 2013;51(5):938-49.
[10] Shim HJ, Go G, Lee H, Choi SW, Won JH. Influence of Visual Deprivation on Auditory Spectral Resolution, Temporal Resolution, and Speech Perception. Frontiers in Neuroscience. 2019;13(1200).
[11] Cornell Kärnekull S, Arshamian A, Nilsson ME, Larsson M. From Perception to Metacognition: Auditory and Olfactory Functions in Early Blind, Late Blind, and Sighted Individuals. Front Psychol. 2016;7:1450.
[12] Tompa, R. The plastic fantastic brain: Why losing one sense rewires others. Fred Hutch News Services. 2016
[13] Blindekuh. About blindekuh: Lights out – let the experience begin.
[14] Spence C, Piqueras-Fiszman B. Dining in the dark. The Psychologist. 2012.
[15] Yelp Inc. “Yelp Edinburgh's January Elite Event: Dining in the Dark @ Edinburgh Larder Bistro” 2013. Available from: https://www.flickr.com/photos/yelp/8406123663/in/photostream/
[16] Castaldi E, Lunghi C, Morrone MC. Neuroplasticity in adult human visual cortex. Neuroscience & Biobehavioral Reviews. 2020;112:542-52.
[17] Norman LJ, Dodsworth C, Foresteire D, Thaler L. Human click-based echolocation: Effects of blindness and age, and real-life implications in a 10-week training program. PLOS ONE. 2021;16(6):e0252330.
Comments